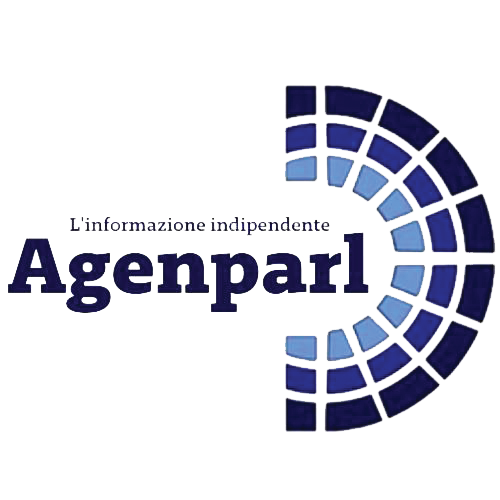
(AGENPARL) – ven 22 marzo 2024 *Source*: Tokyo Institute of Technology
*Immediate release:* March 22, 2024
*Headline*: Discovery of a Hidden Quantum Critical Point in Two-Dimensional
Superconductors
*Sub-headline: * Thermoelectric Effect Reveals Full Picture of Fluctuations
in Superconductivity
*Body: *(Tokyo, March 22) Weak *fluctuations in superconductivity* 1, a
precursor phenomenon to superconductivity, have been successfully detected
by a research group of Tokyo Institute of Technology (Tokyo Tech). This
breakthrough was achieved by measuring the *thermoelectric effect* 2 in
superconductors over a wide range of magnetic fields and over a wide range
of temperatures from much higher than the superconducting transition
temperature to very low temperatures near absolute zero. This revealed the
full picture of fluctuations in superconductivity with respect to
temperature and magnetic field, and demonstrated that the origin of the
anomalous metallic state in magnetic fields, which has been an unsolved
problem in the field of *two-dimensional superconductivity* 3 for 30 years,
is the existence of a *quantum critical point* 4 where quantum fluctuations
are at their strongest.
*? Background*
A superconductor is a material in which electrons pair up at low
temperatures, resulting in zero electrical resistance. It is used as a
material for powerful electromagnets in medical MRI and other applications.
They are also deemed crucial as tiny logic elements in quantum computers
that operate at cryogenic temperatures, and there is a need to elucidate
the properties of superconductors at cryogenic temperatures when they are
microminiaturized.
Atomically thin two-dimensional superconductors are strongly affected
by fluctuations
and thus exhibit properties that differ significantly from those of thicker
superconductors. There are two types of fluctuations: thermal (classical),
which is more pronounced at high temperatures, and quantum, which is more
important at very low temperatures, and the latter causes a variety of
interesting phenomena. For example, when a magnetic field is applied
perpendicularly to a two-dimensional superconductor at absolute zero and
increased, a transition occurs from zero resistance superconductivity to an
insulator with localized electrons. This phenomenon is called the magnetic
field-induced superconductor-insulator transition and is a typical example
of a *quantum phase transition* 4 caused by quantum fluctuations. However,
it has been known since the 1990s that for samples with relatively weak
localization effects, an anomalous metallic state appears in the
intermediate magnetic field region where the electrical resistance is
several orders of magnitude lower than the normal state. The origin of this
anomalous metallic state is thought to be a liquid-like state in which
magnetic flux lines (Fig. 1 left) that penetrate into the superconductor
move around due to quantum fluctuations. However, this prediction has not
been substantiated because most previous experiments on two-dimensional
superconductors have used electrical resistivity measurements that examine
the voltage response to current, making it difficult to distinguish between
voltage signals originating from the motion of magnetic flux lines and
those originating from the scattering of normal-conducting electrons.
A research team led by Assistant Professor Koichiro Ienaga and Professor
Satoshi Okuma of the Department of Physics, School of Science at Tokyo Tech
reported in *Physical Review Letters*
in
2020 that quantum motion of magnetic flux lines occurs in an anomalous
metallic state by using the thermoelectric effect, in which voltage is
generated with respect to heat flow (temperature gradient) rather than
current. However, to further clarify the origin of the anomalous metallic
state, it is necessary to elucidate the mechanism by which the
superconducting state is destroyed by quantum fluctuation and transitions
to the normal (insulating) state. In this study, they performed
measurements aimed at detecting the superconducting fluctuation state
(center of Fig. 1), which is a precursor state of superconductivity and is
thought to exist in the normal state.
*? Research results*
In this study, a molybdenum-germanium (Mo*x*Ge1-*x*) thin film with an
*amorphous
structure* 5, known as a two-dimensional superconductor with uniform
structure and disorder, was fabricated and used. It is 10 nanometers thick
(one nanometer is one billionth of a meter) and promises to have the
fluctuation effects characteristic of two-dimensional systems. Since
fluctuation signals cannot be detected by electrical resistivity
measurements because they are buried in the signal of normal-conducting
electron scattering, we performed thermoelectric effect measurements, which
can detect two types of fluctuations: (1) superconducting fluctuations
(fluctuations in the amplitude of superconductivity) and (2) magnetic flux
line motion (fluctuations in the phase of superconductivity). When a
temperature difference is applied in the longitudinal direction of the
sample, the superconducting fluctuations and the motion of the magnetic
flux lines generate a voltage in the transverse direction. In contrast,
normal electron motion generates voltage primarily in the longitudinal
direction. Especially in samples such as amorphous materials, where
electrons do not move easily, the voltage generated by electrons in the
transverse direction is negligible, so the fluctuation contribution alone
can be selectively detected by measuring the transverse voltage (Fig. 1,
right).
The thermoelectric effect was measured in a variety of magnetic fields and
in a variety of temperatures ranging from much higher than the
superconducting transition temperature of 2.4 K (Kelvin) to very low
temperature of 0.1 K (1/3000 of 300 K, the room temperature), which is
close to absolute zero. That reveals that superconducting fluctuations
survive not only in the liquid region of the magnetic flux (dark red region
in Fig. 2), where superconducting phase fluctuations are more pronounced,
but also over a wide temperature-magnetic field region farther outwards
that is considered to be the normal-state region, where superconductivity
is destroyed (the high-temperature and high-magnetic field region above the
upper convex solid line in Fig. 2). Notably, the crossover line between
thermal (classical) and quantum fluctuations was successfully detected for
the first time (thick solid line in Fig. 2). The value of the magnetic
field when the crossover line reaches absolute zero likely corresponds to
the quantum critical point where quantum fluctuations are strongest, and
that point (white circle in Fig. 2) is clearly located inside the magnetic
field range where an anomalous metallic state was observed in the
electrical resistance. It was not possible to detect the existence of this
quantum critical point from electrical resistivity measurements until now.
This result reveals that the anomalous metallic state in a magnetic field
at absolute zero in two-dimensional superconductors, which has remained
unresolved for 30 years, originates from the existence of the quantum
critical point. In other words, the anomalous metallic state is a
broadened quantum
critical ground state for the superconductor-insulator transition.
*? Social impact*
The thermoelectric effect measurements obtained for amorphous conventional
superconductors can be regarded as standard data for the thermoelectric
effect on superconductors, since they capture purely the effect of
fluctuations in superconductivity without the contribution of normal-state
electrons. The thermoelectric effect is important in terms of its
application to electric cooling systems, etc., and there is a need to
develop materials that exhibit a large thermoelectric effect at low
temperatures to extend the limit of cooling temperatures. Anomalously large
thermoelectric effects have been reported at low temperatures in certain
superconductors, and comparison with the present data may provide a clue to
their origin.
*? Future development*
Of academic interest that will be developed in this study is demonstrating the
theoretical prediction that in two-dimensional superconductors with
stronger localization effects than the present sample, the magnetic flux
lines will be in a *quantum condensed state*6. Moving forward, we plan to
deploy experiments using the methods of this study with the aim of
detecting them.
The results of this study were published online in *Nature Communications*
on March 16th, 2024.
*[Terms]*
(1) *Fluctuations in superconductivity: *The strength of
superconductivity is not uniform and fluctuates in time and space. It is
normal for thermal fluctuations to occur, but near absolute zero, quantum
fluctuations occur based on the quantum mechanical uncertainty principle.
(2) *Thermoelectric effect: *An effect of exchanging thermal and
electrical energy. A voltage is generated when a temperature difference is
applied, while a temperature difference is produced when a voltage is
applied. The former is being studied for application as a power generation
device and the latter as a cooling device. In this study, it is used as a
method to detect fluctuations in superconductivity.
(3) *Two-dimensional superconductivity:* A very thin superconductor.
When the thickness becomes thinner than the distance between the pairs of
electrons responsible for superconductivity, the effect of fluctuations in
superconductivity becomes stronger, and the properties of the
superconductors are very different from those of thicker superconductors.
(4) *Quantum critical point, quantum phase transition: *A phase
transition that occurs at absolute zero when a parameter such as a magnetic
field is changed is called a quantum phase transition, and is distinguished
from a phase transition caused by a temperature change. The quantum
critical point is the phase transition point where a quantum phase
transition occurs and where quantum fluctuations are strongest.
(5) *Amorphous structure: *A structure of material in which
atoms are arranged in an irregular manner and which has no crystalline
structure.
(6) *Quantum condensed state: *A state in which a large number
of particles fall into the lowest energy state and behave as a singular
macroscopic wave. In the superconducting state, many pairs of electrons are
condensed. Liquid helium also condenses when cooled to 2.17 K, producing a
superfluidity with zero viscosity.
*Reference*
Authors:
Koichiro Ienaga*, Yutaka Tamoto, Masahiro Yoda, Yuki Yoshimura, Takahiro
Ishigami, and Satoshi Okuma
Title:
Broadened quantum critical ground state in a disordered superconducting
thin film
Journal:
*Nature Communications*
DOI:
10.1038/s41467-024-46628-7
Affiliations:
Department of Physics, Tokyo Institute of Technology, Japan
Figure 1 : https://tokyotech.box.com/s/yc4wball1krq1e3oot6qm06gjmxac3q5
Figure 1. title: *Two Types of Fluctuaitions in Superconductors and their
Detection by Thermoelectric Effect Measurement*
Figure 1. caption: (Left) In a magnetic field of moderate magnitude,
magnetic flux lines penetrate in the form of defects accompanied by
vortices of superconducting currents. (Center) Conceptual diagram of the
“superconducting fluctuation” state, a precursor to superconductivity.
Time-varying, spatially non-uniform, bubble-like superconducting regions
are formed. (Right) Schematic diagram of thermoelectric effect measurement.
Magnetic flux line motion and superconducting fluctuations generate a
voltage perpendicular to the heat flow (temperature gradient).
Figure 2: https://tokyotech.box.com/s/squ4huth7s0uw7x6bolwr1p6jlxhce27
Figure 2. title: *Color map of a thermoelectric signal that captures the
fluctuations in superconductivity *
Figure 2. caption: The full picture of fluctuations in superconductivity
has been revealed over a wide magnetic field range and over a wide
temperature range, from much higher than the superconducting transition
temperature to very low temperature of 0.1 K. The existence of a crossover
line between thermal (classical) and quantum fluctuations is demonstrated
for the first time, and the quantum critical point where this line reaches
absolute zero is found to exist inside the anomalous metallic region.
*Contact: *Emiko Kawaguchi, Public Relations Department, Tokyo Institute
*About Tokyo Institute of Technology*
Tokyo Tech stands at the forefront of research and higher education as the
leading university for science and technology in Japan. Tokyo Tech
researchers excel in fields ranging from materials science to biology,
computer science, and physics. Founded in 1881, Tokyo Tech hosts over
10,000 undergraduate and graduate students per year, who develop into
scientific leaders and some of the most sought-after engineers in industry.
Embodying the Japanese philosophy of “monotsukuri,” meaning “technical
ingenuity and innovation,” the Tokyo Tech community strives to contribute
to society through high-impact research.
https://www.titech.ac.jp/english/