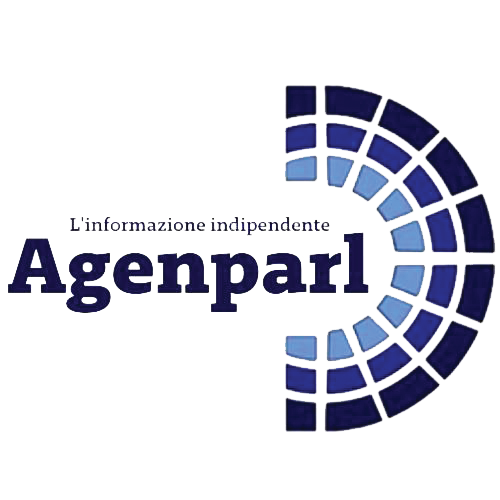
(AGENPARL) – ven 08 aprile 2022 A weekly compendium of media reports on science and technology achievements at Lawrence Livermore National Laboratory. Though the Laboratory reviews items for overall accuracy, the reporting organizations are responsible for the content in the links below.
LLNL Report, April 8, 2022
A gold cylinder known as a hohlraum holds a fuel capsule at its center for fusion experiments at the National Ignition Facility. Target-handling systems precisely position the capsule and cool it to cryogenic temperatures.
[Burning for you](https://physicstoday.scitation.org/doi/10.1063/PT.3.4976)
Inertial fusion requires a thousand-fold compression of matter to ultrahigh densities and temperatures. The sun and other stars use gravity to do the job and fuse hydrogen into helium. To mimic the effect on Earth, scientists at Lawrence Livermore National Laboratory’s National Ignition Facility (NIF) use the world’s most powerful bank of lasers to squeeze isotopes of hydrogen — deuterium and tritium — in a 2-mm-wide capsule.
The facility trains 192 laser beams into a 1-cm-tall, hollow, gold-lined cylinder known as a hohlraum that suspends the capsule inside it. After absorbing UV-laser light, the hohlraum’s interior wall reradiates a flux of soft X-rays. Within 8 nanoseconds, those X-rays accelerate and compress the hydrogen isotopes into a hot spot half the width of a human hair at a temperature of 60 million kelvin and a pressure of 350 GPa (one GPa is equivalent to 9,869 atmospheres of pressure on Earth).
Under the capsule’s surface the hydrogen fuel resides as a thin shell, cooled to 18 K prior to compression.. The colder the fuel is initially, the more compressible it is — and hence the hotter and denser it becomes. The fuel’s own inertia provides enough delay between the implosion and its sudden deceleration for the strong nuclear force to convert a small fraction of isotope pairs into neutrons and helium nuclei, or alpha particles.
[Read More](https://physicstoday.scitation.org/doi/10.1063/PT.3.4976)
The multiscale model incorporates both microstructural (left) and atomistic (right) simulations to understand barriers to ion transport in solid-state battery materials. Image by Brandon Wood, Tae Wook Heo and Sabrina Wan/LLNL.
[Breaking down barriers](https://semiengineering.com/microstructural-impacts-on-ionic-conductivity-of-oxide-solid-electrolytes-from-a-combined-atomistic-mesoscale-approach/)
Solid electrolytes may overcome key technological hurdles associated with the narrow electrochemical and thermal stability of conventional lithium (Li)-ion and sodium (Na)-ion batteries.
However, many solid electrolytes — ceramics in particular — also suffer from poor cycling issues and limitations in their ability to efficiently transport ions. These limitations often stem from interfaces and other features that make up the microstructure of the material, which in turn depends on how it is processed.
Lawrence Livermore National Laboratory scientists in collaboration with San Francisco State University and Penn State have developed a broad suite of multiscale simulation capabilities to help identify, assess and overcome microstructural impacts on ion transport in solid electrolytes
[Read More](https://semiengineering.com/microstructural-impacts-on-ionic-conductivity-of-oxide-solid-electrolytes-from-a-combined-atomistic-mesoscale-approach/)
A cryogenic target used for experiments producing burning-plasma conditions. Photo by Jason Laurea/LLNL.
[The search for the holy grail](https://www.thedailybeast.com/nuclear-fusion-might-finally-be-ready-for-its-breakthrough-moment)
If you’ve ever been outside during the day, you’ve seen a fusion reactor — though you’re not supposed to stare directly at it. The sun is our fusion reactor in the sky. It’s constantly fusing hydrogen atoms to create helium, and this process generates a massive amount of energy, which produces the light and heat that make life on Earth possible.
Here on the surface of this planet, scientists are trying to replicate this process to revolutionize how we generate clean electricity. Fusion researchers believe this technology could be the key to meeting our vast energy needs.
Since fusion was discovered in 1934, we’ve been trying to harness the energy created by nuclear fusion to produce carbon-free electricity — a tremendous amount of it. The goal of fusion research is to achieve what’s called “ignition,” which is when the fusion reaction creates more energy than was used to spark the reaction.